<< View SCS Blog
Ideal Coatings for Medical Devices
Parylene is a polymeric material that is commonly applied as a conformal coating layer in electronic applications. Parylene conformal coatings are used to provide barrier protection from environmental elements and/or to provide dielectric isolation in charged conditions. They offer pinhole-free layers with low water permeability, high flexibility and high mechanical strength. While Parylene coatings are frequently applied to electronic circuitry and sensors, they also have impeccable properties that are beneficial for use on other medical surfaces.
Use of Parylene as a Packaging Material for Biomedical Applications
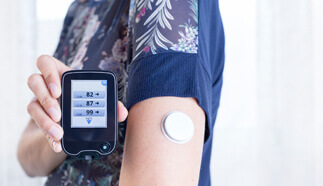
Medical devices are often exposed to the biochemical environment of the living organisms and are prone to chemical attack by various body fluids upon prolonged contact with human tissue at 37 °C and between pH 5.4–7.4 [1]. As a result, there is an inherent need for hermetic packaging that does not trigger immune responses. Additionally, isolating electronic components and systems is needed for a variety of medical applications.
Parylenes have been widely investigated for use in such applications. Materials used in medical systems packaging will interface with living tissue and biochemical liquids. Parylenes N, C, Parylene HT® and ParyFree® comply with the FDA ISO 10993 regulations for biomedical use for medical applications microfluidic devices and medical implants (stents, needles, etc.). As such, they can also be used on biomedical devices that include skin patches that contact with the skin for data collection as well as other sensors including pressure sensors for heartbeat, thermal sensors for body temperature, chemical sensors for sweat analysis, acoustic sensors in cochlear implants, etc. Medical applications ranging from neural prosthetic applications, catheters and stents to skin patches makes use of Parylene conformal coatings [2]–[5].
Prior Studies on the Biocompatibility of Parylene
To assess Parylene barrier properties to prevent biocorrosion, the scientific community has conducted related investigations. Coatings for improving corrosion resistance of stainless steel 316L implant materials investigated the effect of bodily fluids on the 2 um thick Parylene N layer using Hanks solution [1], an artificial salts mixture including blood serum and tissue extracts. The images below demonstrate that Parylene behaves as an ideal candidate for an implant conformal coating. In the study, a deterioration mechanism was proposed for the cracked film. It was reported that the deterioration starts from the metal/polymer interface and progress towards the outer surface resulting in cracks as seen in the image.

(1) Cross-section imaging of of the polymer coating on stainless steel (2) SEM images of the polymer coated stainless steel before exposure (3) After 7 days of exposure to Hanks solution (4) Hanks solution with H2O2 (Image Ref: [1])
A comparative study on Parylene N and C revealed that for Parylene C coatings, the critical load for initial cracks is 3–5 times higher and the total metal ions release is reduced by a factor of 3 compared to Parylene N [7].
A five months study on Parylene C as a packaging material on test chips to protect CMOS circuitry kept at 55°C in isotonic saline soak tests has shown that the conformal coating was not affected by the soak test [8].
In yet another study making use of 25 μm thick Parylene C as a packaging material for neuro sensor implants (in vivo and in vitro), a preliminary assessment of the stability for in vivo implantation showed that Parylene C is an ideal packaging material as a flexible substrate for bio-medical implants [9] . It has a well-known and established deposition process which also offers compatibility with standard microfabrication processes for use with MEMS-based implants and skin patches. Its ISO class VI biocompatibility makes it a particularly great candidate as does its transparency and flexibility which offers ease of surgical manipulation.
References:
[1] M. Cieślik, K. Engvall, J. Pan, and A. Kotarba, “Silane–Parylene coating for improving corrosion resistance of stainless steel 316L implant material,” Corros. Sci., vol. 53, no. 1, pp. 296–301, Jan. 2011, doi: 10.1016/j.corsci.2010.09.034.
[2] L. Wei, A. Lakhtakia, A. P. Roopnariane, and T. M. Ritty, “Human fibroblast attachment on fibrous Parylene-C thin-film substrates,” Mater. Sci. Eng. C, vol. 30, no. 8, pp. 1252–1259, Oct. 2010, doi: 10.1016/j.msec.2010.07.003.
[3] P.-J. Chen et al., “Implantable micromechanical Parylene-based pressure sensors for unpowered intraocular pressure sensing,” J. Micromechanics Microengineering, vol. 17, no. 10, pp. 1931–1938, Aug. 2007, doi: 10.1088/0960-1317/17/10/002.
[4] R. Caldwell, M. G. Street, R. Sharma, P. Takmakov, B. Baker, and L. Rieth, “Characterization of Parylene-C degradation mechanisms: In vitro reactive accelerated aging model compared to multiyear in vivo implantation,” Biomaterials, vol. 232, p. 119731, Feb. 2020, doi: 10.1016/j.biomaterials.2019.119731.
[5] C.-C. Wu et al., “A pliable and batteryless real-time ECG monitoring system-in-a-patch,” in VLSI Design, Automation and Test(VLSI-DAT), Apr. 2015, pp. 1–4, doi: 10.1109/VLSI-DAT.2015.7114521.
[6] M. Cieślik et al., “Engineering of bone fixation metal implants biointerface—Application of Parylene C as versatile protective coating,” Mater. Sci. Eng. C, vol. 32, no. 8, pp. 2431–2435, Dec. 2012, doi: 10.1016/j.msec.2012.07.018.
[7] M. Cieślik, M. Kot, W. Reczyński, K. Engvall, W. Rakowski, and A. Kotarba, “Parylene coatings on stainless steel 316L surface for medical applications — Mechanical and protective properties,” Mater. Sci. Eng. C, vol. 32, no. 1, pp. 31–35, Jan. 2012, doi: 10.1016/j.msec.2011.09.007.
[8] M. Kazemi et al., “A test microchip for evaluation of hermetic packaging technology for biomedical prosthetic implants,” in The 26th Annual International Conference of the IEEE Engineering in Medicine and Biology Society, San Francisco, CA, USA, 2004, vol. 4, pp. 4093–4095, doi: 10.1109/IEMBS.2004.1404142.
[9] A. Lecomte, A. Degache, E. Descamps, L. Dahan, and C. Bergaud, “In vitro and in vivo biostability assessment of chronically-implanted Parylene C neural sensors,” Sens. Actuators B Chem., vol. 251, pp. 1001–1008, Nov. 2017, doi: 10.1016/j.snb.2017.05.057.
[10] W. R. Dolbier and W. F. Beach, “Parylene-AF4: a polymer with exceptional dielectric and thermal properties,” J. Fluor. Chem., vol. 122, no. 1, pp. 97–104, Jul. 2003, doi: 10.1016/S0022-1139(03)00100-3.